Electrical power distribution necessitates comprehensive solutions. Totally Integrated Power (TIP) offers assistance in devising appropriate solutions. It includes software tools and support for planning and configuration, along with a fully harmonized portfolio of products and systems for seamless power distribution, extending from medium-voltage switchgear to the terminal circuit.
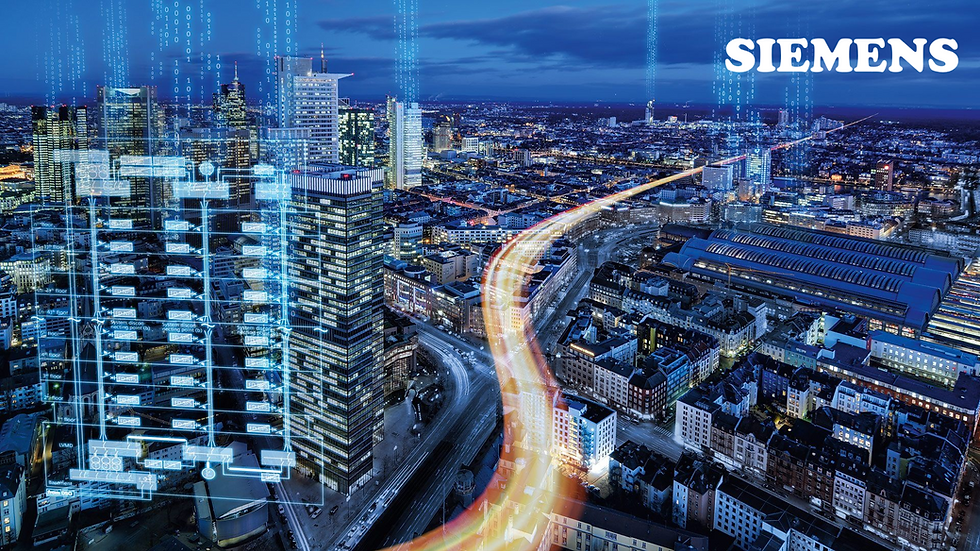
With TIP Siemens renders support to meet requirements such as:
Simplification of operational management by a transparent, simple network topology
Low network losses, for example by medium-voltage-side energy transport to the load centres
High reliability of supply and operational safety of the installations, even in the event of individual equipment failures (redundant supply, selectivity of the network protection, and high availability)
Easy adaptation to changing load and operational conditions
Low operating costs thanks to maintenance-friendly equipment
Sufficient transmission capacity of the equipment under normal operating conditions as well as in fault conditions to be controlled
Good quality of the power supply, meaning few voltage changes due to load fluctuations with sufficient voltage symmetry and few harmonic distortions in the voltage
Observance of valid IEC/EN/VDE regulations as well as project-related regulations for special installations.
Qualified planning of a network concept which considers the above-mentioned aspects is the key to the efficiency of electric power supply. Network concepts must always be assessed in the context of their framework parameters and project goals.
Fig. 1/1: Tasks of network planning and configuration
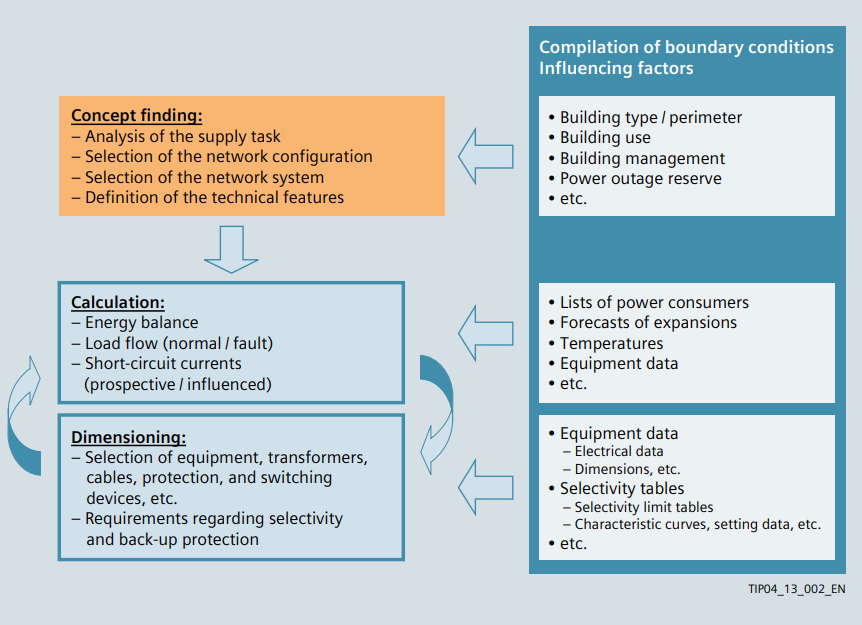
Siemens TIP supports electrical planners in network planning and configuration (see Fig. 1/1) with a wide range of services. Our TIP contact partners (please find their contact data on the Internet (at www.siemens.com/tip-cs/contact) also make use of their personal contact to you to present you with planning tools such as SIMARIS design, SIMARIS project and SIMARIS curves.
Besides planning manuals, Siemens also offers application manuals, which describe the planning specification of certain property types like high-rise buildings, hospitals, or data centers, and more network calculation tools like PSS®SINCAL.
The Table of Contents:
1. Requirements for Electrical Networks in Buildings
When electrical networks are planned, largely ambivalent requirements of the three project “life stages” must be considered:
Investment
Installation
Operation
Tab. 1/1 renders a assessment of the expense incurring in these different “life stages”
Further influencing factors
The essential properties of a network are determined by the following requirements:
Usage/ consumers or respective purpose of power distribution, which means power balance, power density, and load centres (see Tab. 1/2)
Architecture, for example, low-rise or high-rise building
Operating and environmental conditions
Official regulations / statutory provisions such as occupational safety and health acts, building authorities
By the supplying electricity utility company
Technical specifications with regard to voltage, shortcircuit power, approval of maximum connected load, permissible technology
Use of power management, in order to profitably operate the network within the given tariff options.
Tab. 1/1: Relation between expense and life stages of a project

Tab. 1/2: Examples of various areas of use and their impact on electrical networks and equipment
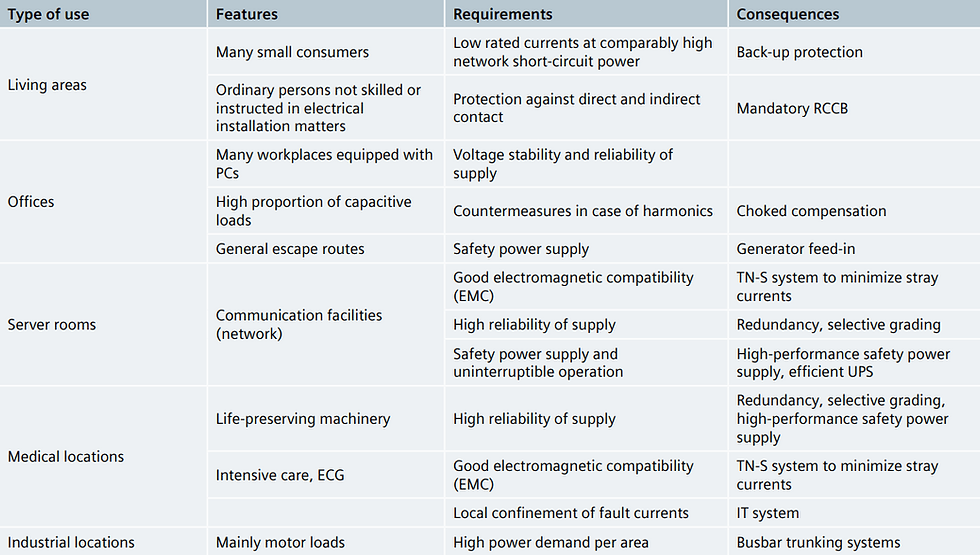
2. Estimation of Power Demand
The basis for planning and sizing power distribution is knowing the equipment to be connected and the resulting total power demand. Besides the power demand of large machinery (motors, pumps, etc.), the demand of individual functional areas (office, parking, shop, etc.) must be ascertained (Tab. 1/3 and Tab. 1/4).
The simultaneity factors can be used when the individual loads for a building or a room / functional area are summed up.
Tab. 1/3: Average power demand of buildings according to their type of use
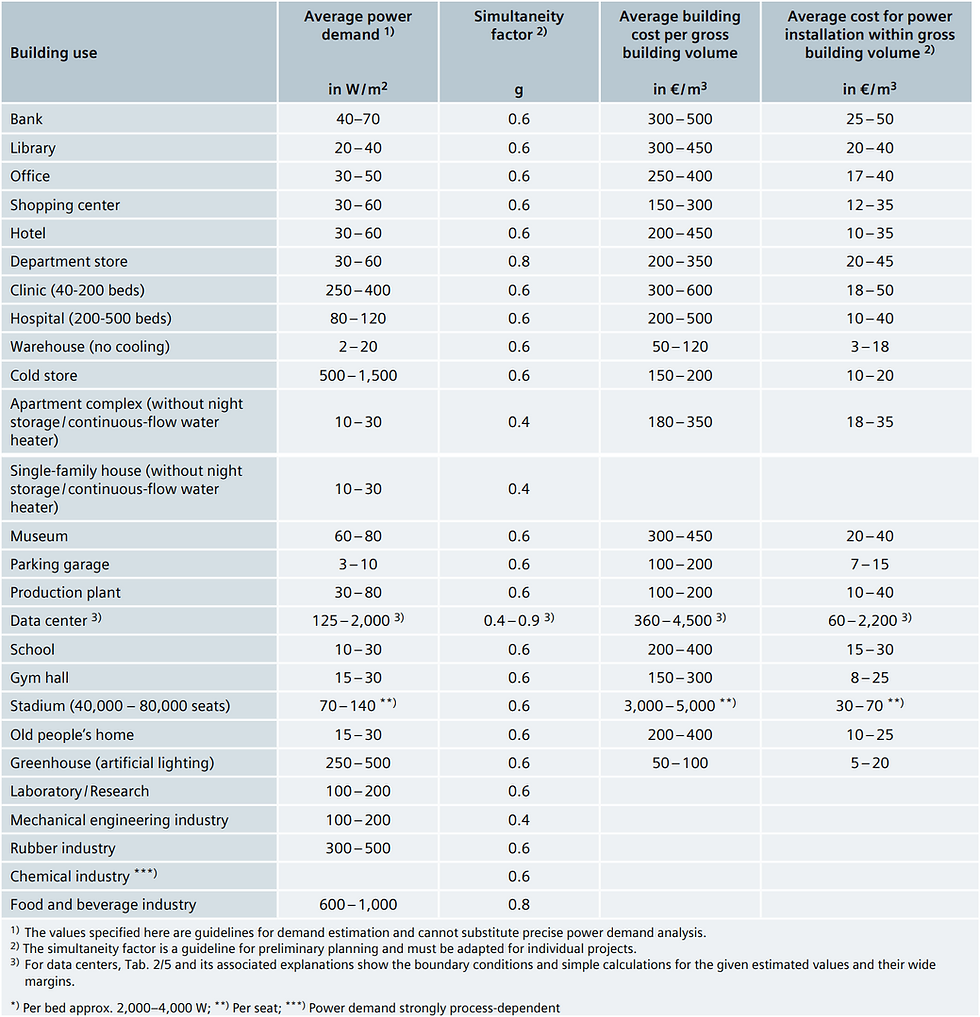
Tab. 1/4: Average power demand of various functional/building areas
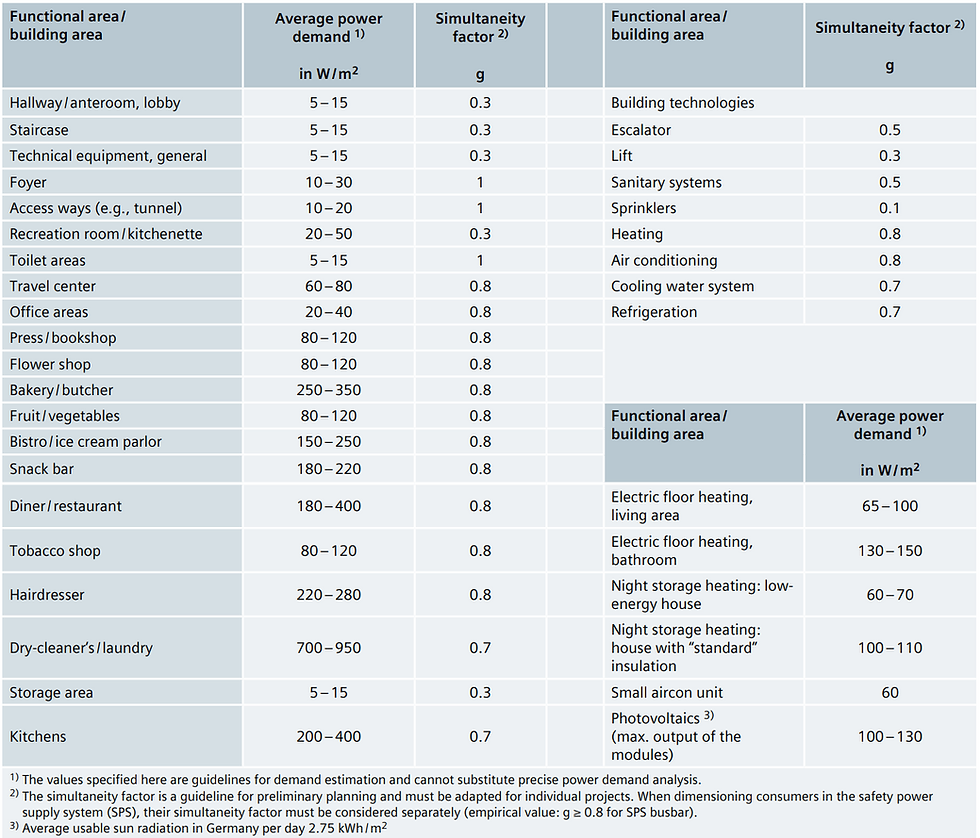
2.1 Special Consideration of the Cost Situation for a Data Center
For a data centre, there are a number of factors influencing, among other things, the specific power demand. Important aspects which result in a wide bandwidth of the estimations of power demand, simultaneity factor, and specific costs are as follows:
Differentiation between a self-contained building (data centre) or the ICT areas in a building
Different technologies for air conditioning and power supply influence space requirements and energy efficiency
Requirements as to availability determine the redundancy and safety systems.
The following assumptions are to be made for data-specific cost estimations:
An area-specific power demand of 125 to 1,500 W/m2 is assumed for a self-contained data centre (DaC in Tab. 2/5). The low value suggests a large space required for information technology and infrastructure (for example owing to high redundancies), whereas the high value suggests a high packing density of servers in the racks, and modern cooling and power supply systems
An area-specific power demand of 500 to 2,000 W/m2 for rooms containing information technology in infrastructure buildings (IT room in Tab. 2/5). These values slightly differ from the ones mentioned above, since infrastructure components can be shared in the building.
The “Tier” structure (with ascending requirements I to IV) of the Uptime Institute, as described in [4], is used as a basis in connection with availability and the redundancy conditions upon which availability is founded. (n+1) redundancy of Tier IV results in approximately 2.5-fold costs for infrastructure components compared to Tier I without redundancy. The influence of the redundancy requirements placed on the specific space required is already taken into account in the first two items outlined here
For the list of costs shown in the second part of Tab. 1/5, the installation components are summed up according to the cost group 440 – Power Installations, listed in DIN 276-1. The following are considered:
441 – High and Medium-Voltage Systems (Switchgear, Transformers) – 442 – Embedded Power Generating Systems
443 – Low-Voltage Switchgear
444 – Low-Voltage Installation Systems
445 – Lighting Systems
446 – Lightning Protection and Earthing Systems
The simultaneity factor for data centers, as shown in Table 2/3, varies from 0.4 to 0.9 based on the infrastructural environment and redundancy capabilities. With a (2n+1) redundancy configuration (refer to chapter 5), the appropriate simultaneity factor ranges from 0.4 (when n = 2) to 0.5 (for significantly large values of n). Conversely, in the absence of redundancy, the data center can have a much higher simultaneity factor.
Tab. 1/5: Data centre (DaC) power demand dependent on the concept for redundancy and infrastructure
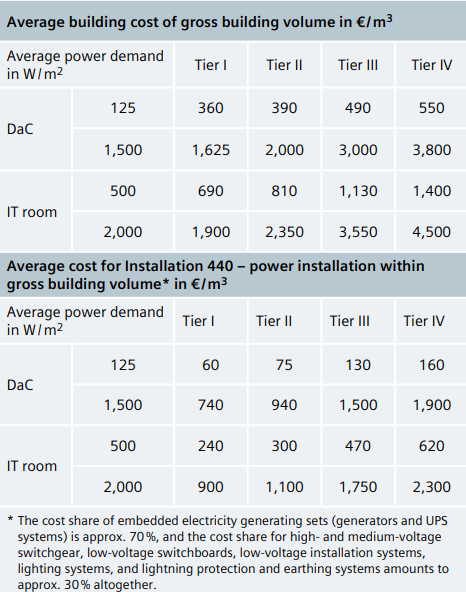
3. Estimation of a Concrete Value for the Power Demand from the Given Margins
The values for the average power demand in Tab. 1/3 and Tab. 1/4 cover a vast bandwidth of different prerequisites. When estimating the total power demand for the project to be planned, the individual margins of building types, functional areas, and rooms must be substantiated. For this purpose, we provide an estimation procedure with various calibration factors below as a simple help.
Note: A similar procedure with efficiency factors is also used in EN 15232-1. In this context, a classification takes place. The division into classes A, B, C, D (Tab. 1/6), for example, applies to the systems used for building automation and control (BAC) and technical building management (TBM). The individual efficiency factors in EN 15232-1 (Tab. 1/7) refer to the mean values for the power demand of the BAC/TBM building systems for the electrical power demand.
To obtain an estimated value within the power demand intervals given in Tab. 1/3 and Tab. 1/4, six calibration factors with values between 0 and 1 are introduced. The individual factors are added and averaged, providing a total factor, also between 0 and 1.
For our simple calculation model, we will limit ourselves to six features considered as equivalent:
Building placement
Room structure
Level of comfort
Air conditioning option
Technical characteristics
BAC/TBM
Certainly, incorporating your own factors as additional boundary conditions is an option. It is crucial for the planner and the customer to coordinate their procedures to ensure the calculations are verifiable. In the model, six calibration factors, each corresponding to one of the six characterization features, determine the building's power demand.
Calibration factor kplc for the building placement
Calibration factor kstruct for the room structure
Calibration factor kcomf for the level of comfort
Calibration factor kclim for the air conditioning options
Calibration factor ktech for the technical characteristics
Calibration factor kBAC/TBM for the BAC/TBM.
Tab. 1/6: Classification of the building automation/control and management systems of a building regarding energy efficiency according to EN 15232-1
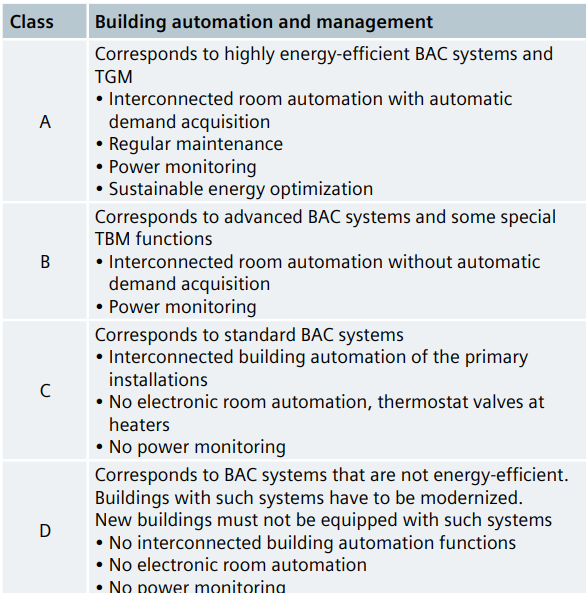
Tab. 1/7: Efficiency factors (electrical) for BAC and TGM functions of non-residential buildings according to EN 15232-1 (standard = class C = 1)
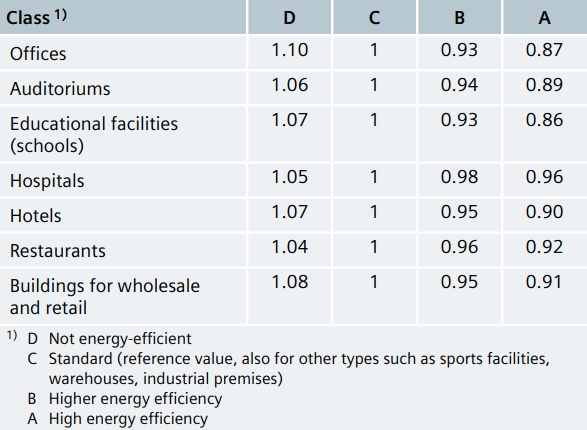
As we do not want to apply any further weighting to the factors, the mean value of the calibration factors can be defined as the total value:

Estimated Power Demand
The established calibration factor ktot and the two limit values for the specific power demand pmin and pmax (e.g., values from Tab. 1/3 and Tab. 1/4) allow determining the mean specific power demand pspec for the entire usable area of a building.

To obtain the total power demand of the building, the mean specific power demand is multiplied by the usable area of the building.
Placement of the building – calibration factor kplc
The location of the building has a fundamental influence on the planning of the power supply.
The following questions can also be used to obtain an estimation:
Do special conditions with regard to adjacent buildings have to be considered?
Which traffic routes and connections can be used?
Which type of power supply is possible and to what extent?
Are there legal boundary conditions that have to be taken into consideration?
Note: Without any local particularities, the placement factor can be set to kplc = 0.5.
Room structure – calibration factor kstruct
Smaller rooms are more efficient to ventilate, and light distribution is enhanced by reflections off the walls and ceiling. The calibration factor may also consider the planned room height. Our estimates, depicted as a curve in Fig. 1/3, account for the fact that smaller spaces often have direct ventilation rather than air conditioning.
Fig. 1/2: Influence of the calibration factors on the specific power

Fig. 1/3: Schematic dependency of the power demand from the building structure demonstrated through a standardized factor kstruct
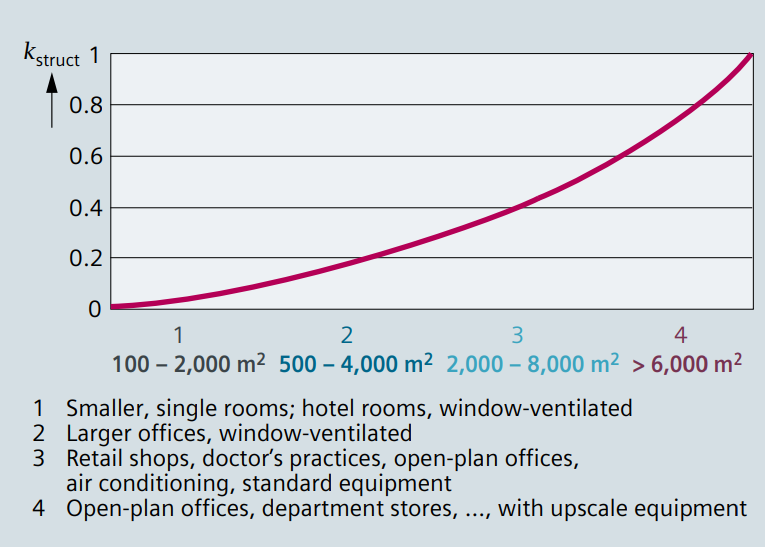
Larger rooms and halls generally have a larger calibration factor kstruct. At this point, we would again like to emphasize that the experience and project knowledge of the planner and the agreement with the customer are decisive in order to determine the factors and thus estimate the costs. Our Siemens TIP contact partners with their background knowledge support electrical planners in specific projects.
Level of comfort and safety equipment – calibration factor kcomf
Making general statements about comfort can be challenging since it greatly depends on the building's usage. Features like good lighting, an audio system, and a monitoring system are standard in a shopping centre, yet they may be seen as comfort features in office spaces. Conversely, blinds are irrelevant for shop windows but are crucial in hotels and offices.
High-speed lifts for large loads require more power, just as special stagecraft technology and technically sophisticated, medical diagnostic equipment. Control and monitoring systems make buildings safe and are the basis for better user-friendliness.
In the production sector, this factor will often play a subordinate part. If one factor is neglected, the number of factors must be reduced accordingly in the denominator of the above equation.
Air conditioning – calibration factor kclim
When considering the air conditioning of a building, it is important to account for natural ventilation, the efficiency of the cooling equipment, and ways to reduce solar radiation without compromising the lighting conditions within the rooms. In Germany, the Association of German Engineers (VDI) has addressed the building-specific power requirements for air ventilation and cooling in the VDI 3807-4 guideline.
The data for the specific installed load in offices, hotel rooms, kitchens, data centers, theaters, department stores, parking garages, and so on, across various demand classes from "very high" to "very low," has been translated into a curve for calibration factors (Fig. 2/4). The overlay of numerous individual curves reveals that only uses with a high cooling demand, like data centers and kitchens, exhibit a marginally different curve profile.
Computer rooms, which are better planned without windows, generally require more expensive air conditioning – constant temperature and humidity – although there is little effect from solar radiation. It should also be noted that the air conditioning depends on the room structure and the comfort requirements.
Technical characteristics – calibration factor ktech
Even when the functionality of the technical building equipment has been defined, the difference in the technical constructions is significant. High-speed lifts require higher starting currents than slower lifts, fans with EC motors (electronically controlled) save power, modern light fittings reduce the power demand, and the efficiency of many electrical consumers differ greatly from version to version.
Fig. 1/4: Schematic dependency of the power demand for the building's air conditioning
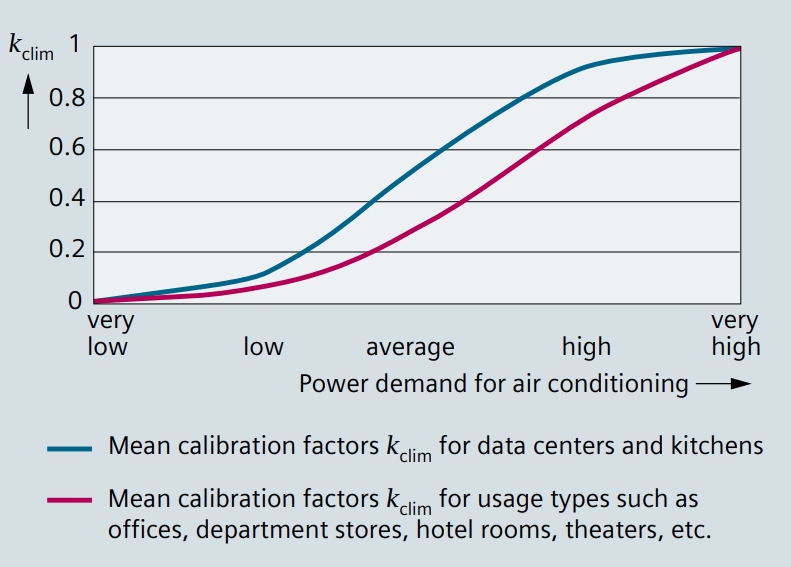
A general classification for the energy efficiency of the technical equipment according to the EN 15232-1 standard is listed in Tab. 1/8. The efficiency factors of EN 15232-1 are transformed in Tab. 1/9 to the desired calibration range between 0 and 1. A distinction is not made for other types (such as sports facilities, warehouses, industrial facilities, etc.) so that the factor of 0.5 is selected for all classes.
Tab. 1/8: Classification of the technical characteristics of a building with regard to energy efficiency
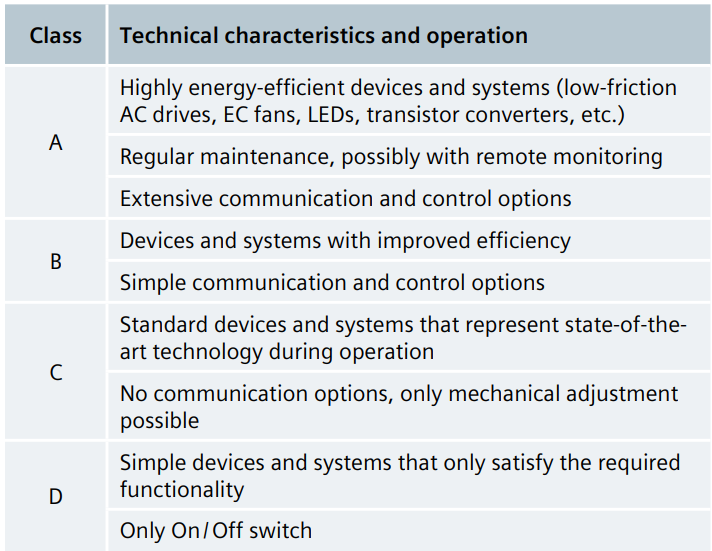
Building management – calibration factor kBAC/TBM
In the same way as for the technical characteristics, standard EN 15232-1 can be used for automation and building management systems (see Tab. 1/9). However, note that energy efficiency class D from EN 15232-1 must not play any role for the planning of BAC/TBM systems in new buildings.
Tab. 1/9: Calibration factors ktech for the technical equipment of a building and kBAC/TBM for building automation/control and technical building management systems, suitable for non-residential buildings

The advantage of our procedure with scaled calibration factors is revealed here. Characterization features can be adapted to state-of-the-art technology through the scaling, and the classification always defined through one's own current experience.
This means that we shift the features of class C of EN 15232-1 (see Tab. 2/6) to our class D and select a description for class A which is even more oriented to efficiency. In the context of BAC / TBM, additional characteristics such as remote monitoring, remote diagnostics, and remote control, as well as analysis tools and integration options as part of the smart grid are requested for the new class A. For the four new classes D, C, B, and A (Tab. 1/10) we then take over the values of the calibration factors kBAC/TBM from Tab. 1/9 accordingly.
Tab. 1/10: Classification of non-residential buildings adapted to new buildings in respect of energy efficiency of BAC/TBM systems
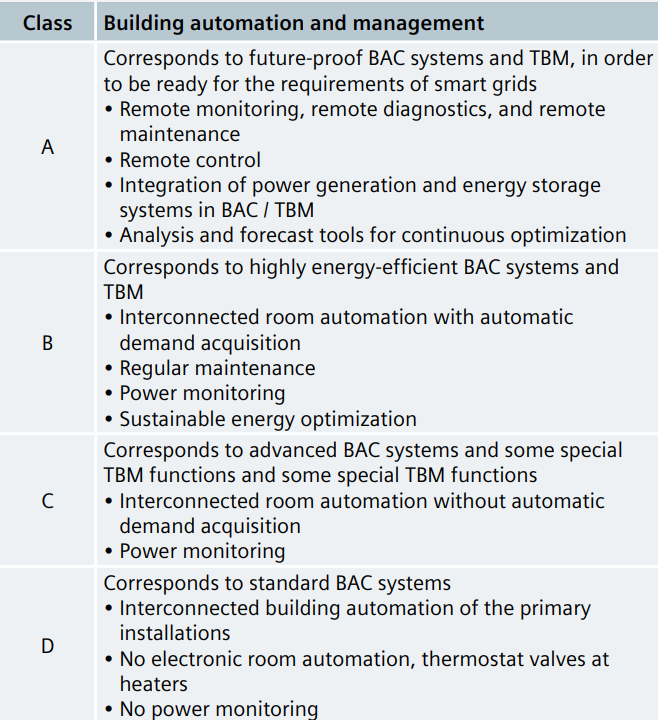
4. Operating Voltages in Supply and Distribution Grids
Different voltages are used to fulfil the different tasks of electric power supply and distribution.
According to international rules, there are initially two voltage groups:
Low voltage (LV): up to and including 1,000 V AC (or 1,500 V DC)
High voltage (HV): above 1 kV AC (or 1.5 kV DC).
Most electrical appliances in households, commercial, and industrial settings operate on low voltage. High voltage is not only utilized for transmitting electrical energy over great distances but also for finely distributed regional delivery to load centres. Transport and regional distribution often require different voltage levels due to the varying tasks and specifications for switching devices and switchgear. This distinction has led to the adoption of the term 'medium voltage' for the voltages used in the regional distribution of electrical energy.
Medium voltage (MV): above 1 kV AC up to and including 52 kV AC; most line operating voltages are within the range of 3 to 40.5 kV (Fig. "/5)
Fig. 1/5: Voltage levels between the power plant and the consumer
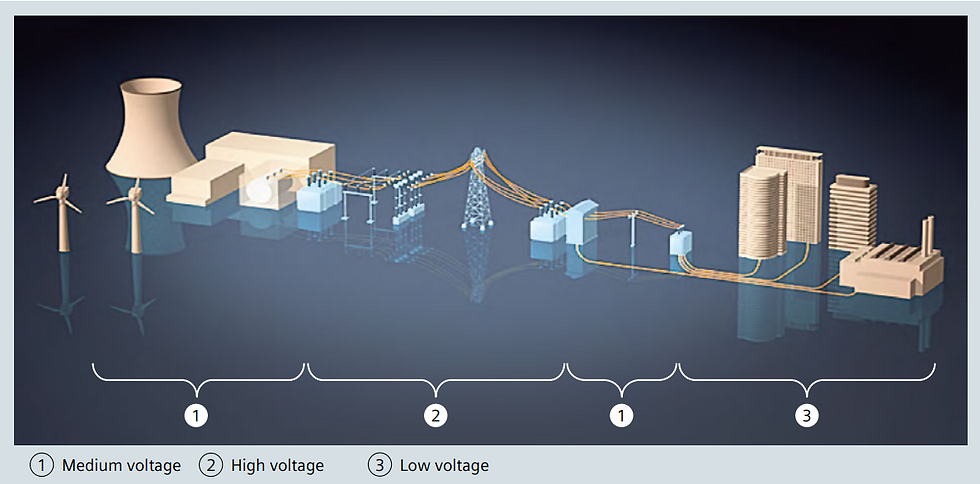
Power plant sites are oriented towards the availability of primary energy sources, cooling systems, and other ambient conditions, and they are therefore mostly located away from load centers. Electric transmission and distribution grids connect power plants with electricity consumers. The grids thus form a supra-regional backbone with reserves to ensure reliability of supply and for balancing load differences. High operating voltages (and therefore low currents) are preferred for power transmission in order to minimize losses. The voltage is then transformed to the usual values of the low-voltage grid in the load centers close to the consumer.
The boundary conditions for selecting the supply voltage and the design of the technical connection points are described in the Technical Connection Conditions (TCC) of the distribution system operator (DSO). In the future, the TCC will be replaced in Germany by the TAR VDE-AR-N 4100 and VDE-AR-N-4110 (Technical Application Rules “TAR Low Voltage” and “TAR Medium Voltage” are available as drafts) as well as by VDE-AR-N 4105 (“TAR Self Generation”).
Among others, this document considers the connection of energy storage units, charging and storage systems for electromobility, or generating plants. Depending on the situation of the DSO with regard to supply density, network short-circuit power and supply quality, an installed capacity between 150 and 1,000 kW may make the connection to the medium-voltage level seem reasonable. Since there is no uniform set of rules, this must be discussed with the responsible DSO during planning.
Dependent on the DSO, a direct connection of the customer to a transformer substation of the DSO (grid level 6 in Tab. 1/11) may be possible in case of a power demand of more than 150 kW (house connection with 250 A), and if a new connection to the grid above 300 or 400 kW needs to be created, a connection to the medium-voltage level (grid level 5) may be permitted. Often, a power factor cos φ is also stipulated (Tab. 1/11).
In the local low-voltage grid, we additionally distinguish between grid level 7a and 7b. Part of grid level 7a are households and small commercial customers with an electricity demand of up to approx. 300 A and 230/400 V feed-in. Industrial and commercial businesses with an electricity demand above 300 A with a 400 V connection are counted as grid level 7b.
In public power supply, the majority of medium-voltage grids are operated in the 10 kV to 30 kV range. The values vary greatly from country to country, depending on the historical technological development and the local conditions. In urban environments, the spatial supply radius of a medium-voltage grid with 10 kV operating voltage is at approx. 5 to 10 km, and in rural areas with 20 kV operating voltage at approx. 10 to 20 km. These are merely guide values. In practice, the supply area strongly depends on local conditions, for example the customer structure (load) and the geographical position.
Apart from the public supply, there are other voltages in industrial plants with medium-voltage grids that depend on the consumers. In most cases, the operating voltages of the installed motors are decisive. Operating voltages between 3 kV and 15 kV are very often used in industrial networks.
The network configuration is determined by the respective supply task, the building dimensions, the number of floors above/below ground, the building use, as well as the building equipment and power density. Typically, areas of different power densities also require different network configurations. In this context, the reliability of supply, and the supply quality of the electric power distribution should be paid special attention to.
An optimal network configuration should meet the following requirements:
Low investment
Straightforward network configuration
High reliability and quality of supply
Low network losses
Favorable and flexible expansion options
Low electromagnetic interference.
The following characteristics must be determined for a suitable network configuration:
Number of feed-in points
Size and type of power sources
Central or distributed installation of the power sources
Type of meshing and size of the power outage reserve
Type of connection to earth and neutral-point connection
Tab. 1/11: Grid level structure in the UCTE grid (UCTE – Union for the Co-ordination of Transmission of Electricity)

5. Type of Feed-in
Electrical energy can be fed into the grid in different ways, determined by its primary function (Tab. 1/12). For normal power supply (NPS):
Direct connection to the public low-voltage grid: in Germany, for example, up to approx. 300 kW (two times 250 A house connection) at 400 / 230 V
Transfer from the medium-voltage grid (up to 52 kV) via public or in-house transformer substations (in Germany mostly with transformers from 0.5 to 2.5 MVA).
For the emergency standby power system (ESPS), power sources are selected based on standards and regulations and as a function of the permissible interruption time:
Generators for general standby operation and/or safety power supply (SPS)
Uninterruptible power supply systems
Static UPS comprising a rectifier/inverter unit with battery or flywheel energy storage for buffering voltage failures
Rotating UPS comprising a motor/generator set with flywheel energy storage or a battery plus rectifier/inverter unit for bridging.
The constellation depicted in Fig. 1/6 with the corresponding description given in Tab. 1/12 has proven itself in infrastructure projects.
Since the circuits for SPS loads must be laid separately, their placement inside the building is relevant for budget considerations.
In Germany, certain statutory regulations and specifications are additionally applicable, which demand the functional endurance of cables and wires in case of fire.
In general, circuits for safety purposes routed through fire-threatened areas must be designed fire-resistant. Never must they be routed through explosion-prone areas. Usually, safety-purpose facilities receive an automatic power supply, whose activation does not depend on operator action. According to IEC 60364-1 (VDE 0100-100), automatic supply is classified by its maximum change-over-time:
Without interruption: automatic supply which can ensure continuous supply during change-over under defined conditions, e.g., with regard to voltage and frequency fluctuations
Very short interruption: automatic supply which is available within 0.15 s
Short interruption: automatic supply which is available within 0.5 s
Mean interruption: automatic supply which is available within 15 s
Long interruption: automatic supply which is available after more than 15 s.
In IEC 60364-5-56 (VDE 0100-560), the following examples of safety installations are given:
Emergency lighting/safety lighting
Fire extinguishing pumps
Fire fighting lifts
Alarm systems such as fire alarm systems, carbon monoxide (CO) alarm systems, and intruder detection systems
Evacuation systems
Smoke evacuation systems
Important medical systems.
Tab. 1/12: Type of feed-in
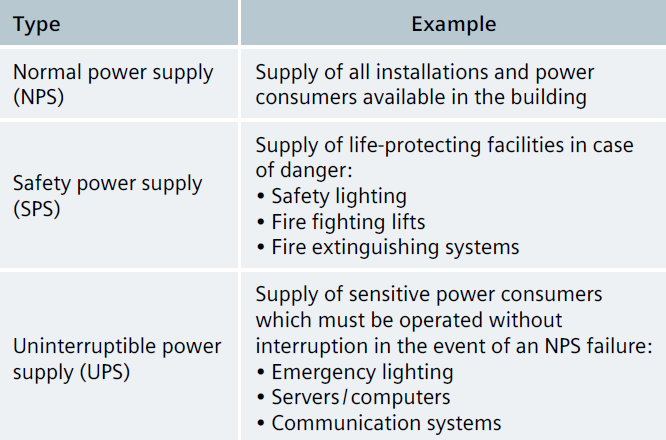
Fig. 1/6: Type of feed-in
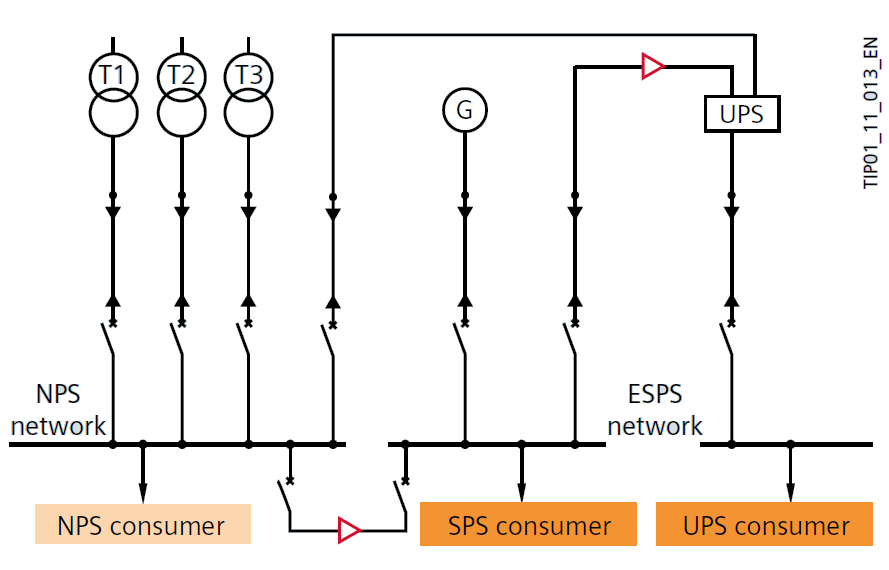
The procedure shown in Fig. 1/7 can be carried out by customers and/or planners for a use-specific classification of different power consumers and the associated corporate-sensitive tasks.
Criteria for the determination of business-critical processes might for example be the following:
Effects on life and health
Protection of important legal interests
Observance of the law and regulations
Loss of the institution’s / company's reputation.
Fig. 1/7: Flowchart for an estimation of NPS, SPS and UPS
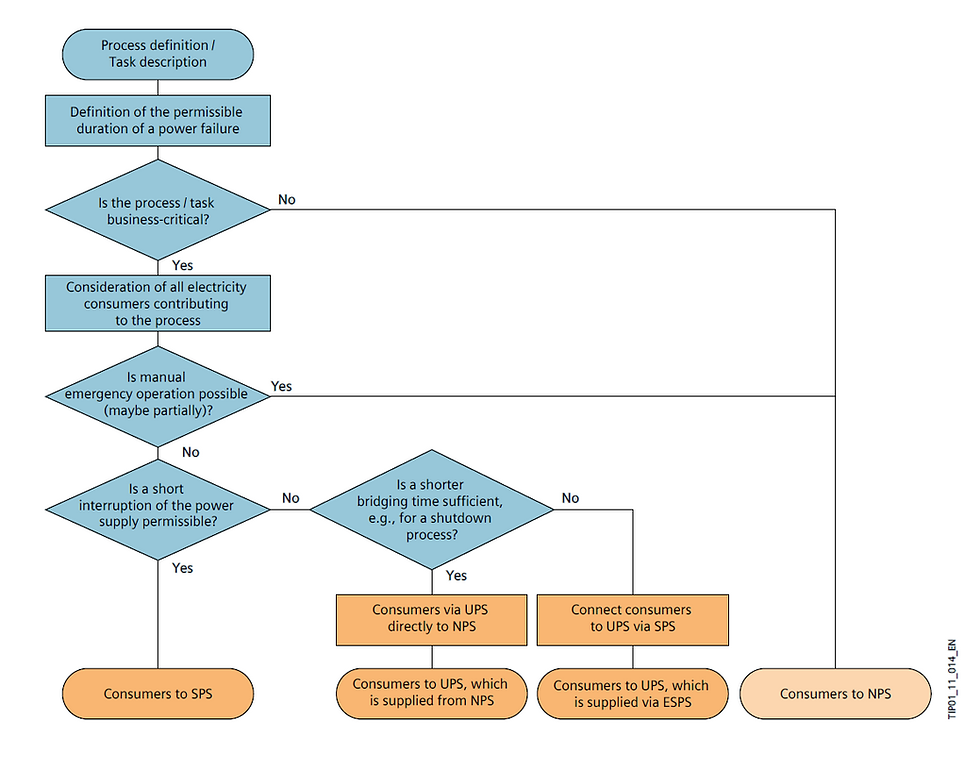
6. Central or Distributed Installation of Low-Voltage Supply
The feed-in design distinguishes between central and distributed feed-in variants in dependency of spatial conditions and the associated load requirements. In case of a central installation, the transformers, which are concentrated in one place, feed into the different power distribution circuits.
In case of a distributed installation, the transformers are placed at load centers, so that they must be spread over a larger area. Fig. 1/8 shows the intrinsic advantages of distributed as compared to central feed-in.
Fig. 1/8: Comparison of feed-in variants with regard to short-circuit current Ik“ and voltage drop Δu

In industrial settings where separate substation rooms are not feasible or desired, transformer load centre substations, such as those depicted in Fig. 1/9, offer a compact and easily installable alternative for distributed power supply.
Fig. 1/9: Load center transformer substation for industrial applications
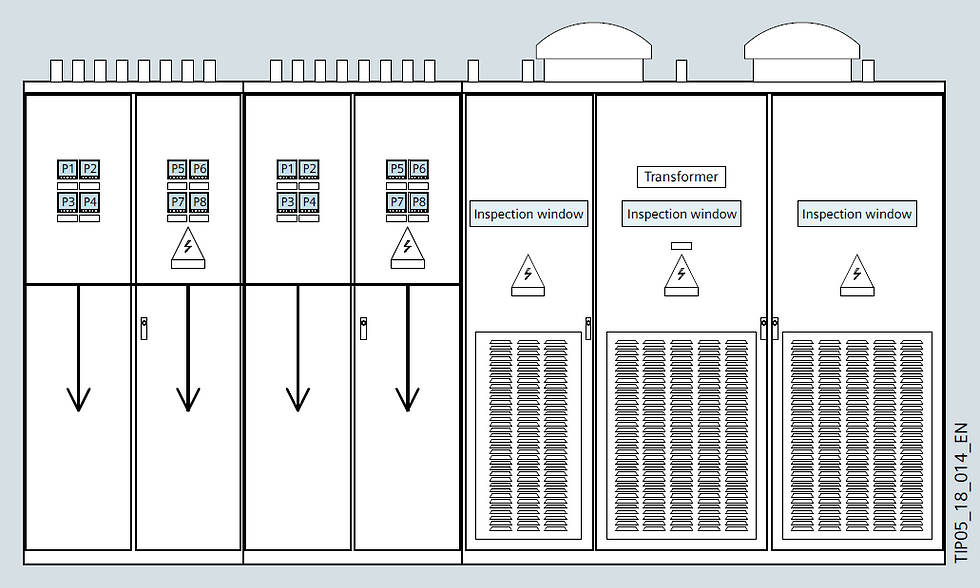
7. Network Configurations
Starting from the type of feed-in, electric power distribution grids or networks can also be distinguished according to their type of meshing. The following basic configurations are distinguished:
Radial networks
Ringed networks
Meshed networks.
The spur-line-fed radial network (Fig. 1/10) is the most simple form. Its advantages lie in easy network monitoring and network protection as well as in fast fault localization and simple operational management. When the expense is doubled, the outcome is a double-spur network.
Fig. 1/10: Radial and ringed network for the connection of distributed load centre substations

Every load center can be reached via two different paths. Switching devices are only closed if necessary. If the requirements placed on reliability of supply are high, each feed-in can be fed from an independent supply network. Due to the fact that the networks are independent from each other, a fault in one network will not affect the other one.
In combination with a ring line as an extension of the spur network (Fig. 2/10), a ringed network can be built up. Dependent on the spatial structures, the investment to be made for an open-type ringed network can be lower or higher than for a spur network. A spur network is advantageous if individual transformers shall handle low-voltage supply in a confined space. A ringed network can be favorable regarding costs of investment if supply is spread out over a larger area with several transformer centers.
In terms of space requirements, power demand coverage, environmental friendliness, and cable costs, the differences between the two network configurations are small. Although ringed networks more often come with shorter cable lengths, the cable cross-section must be higher owing to the transmission of higher capacities from one ring endpoint to the other.
With regard to the costs of network losses, the spur network and the open-type ringed network only differ insignificantly. There are minimal advantages if the ringed network is operated in the closed-type variant. However, protection of the closed ring requires circuit-breakers and
line differential protection or directional protection. These additional costs show up in investments.
In case of a cable fault in an open-type ringed network, all substations downward of the fault location up to the normally open switch will fail. In case of low-voltage-side meshing of the ring-main units, the failure of a large sub-ring could result in overload and disconnection of non-affected, still operable transformers, whereas a cable fault in the spur network merely results in the failure of one station.
Only with a closed-typed ringed network and appropriate protection expense could such a level of reliability be also attained in the ringed network. In addition to this, the closed-type ringed network provides an immediate reserve in case of cable faults, whereas the spur network merely offers a switchover reserve. A single fault with transformer failure can be handled in both networks without interruption if (n-1) redundancy applies to the transformers.
Furthermore, operating a ringed network always requires distributed switching operations which hamper ease of operation. Switching operations for fault localization and actions to attain a defined switching condition in cases of defect are more complicated than with a radial network.
Weather-dependent power feed-in of solar and wind power plants increasingly burdens grids owing to fluctuations which can inadequately be planned only. In line with this, safely connecting or disconnecting parts of the network, together with awareness of the situation, are becoming more and more important.
8. Network Systems according to their Type of Connection to Earth
Suitable network systems according to the type of connection to earth are described in IEC 60364-1 (VDE 0100-100). The type of connection to earth must be selected carefully for the medium- or low-voltage grid, as it has a major impact on the expense required for protective measures (Fig. 1/11). On the low-voltage side, it also influences the system’s electromagnetic compatibility (EMC). From experience, the TN-S system has the best cost-benefit ratio of electricity grids at the low-voltage level.
Fig. 1/11: Systems according to the type of connection to earth in accordance with IEC 60364-1 (VDE 0100-100)
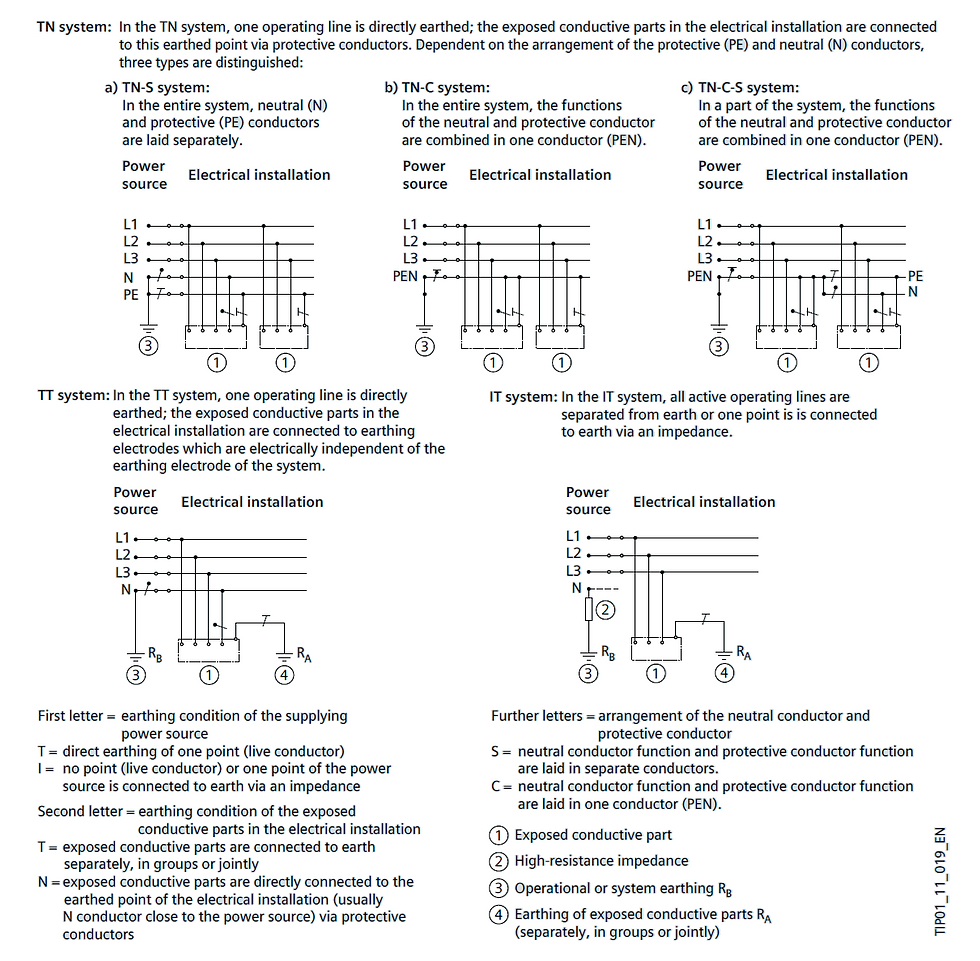
In a TN system, in the event of a short circuit to an exposed conductive part, the main part of the single-phase short-circuit current is not fed back to the power source via a connection to earth, but via the protective conductor. The comparatively high single-phase short-circuit current allows for the use of simple protection devices such as fuses or miniature circuit-breakers, which trip in the event of a fault within the permissible tripping time. In building technology, networks with TN systems are preferably used today.
When using a TN-S system in the entire building, residual currents in the building, and thus an electromagnetic interference by galvanic coupling, can be prevented in normal operation because the operational currents flow back exclusively via the separately laid isolated N conductor.
In case of a central arrangement of the power sources, we always recommend the TN system as a rule. In that, the system earthing is implemented at one central earthing point (CEP), for example in the low-voltage main distribution system, for all sources (Fig. 1/12).
Please note that neither the PEN nor the PE must be switched. If a PEN conductor is used, it is to be insulated over its entire course – this includes the distribution system. The magnitude of the 1-phase short-circuit current directly depends on the position of the CEP.
Caution: In extensive supply networks with more than one splitter bridge, stray short-circuit currents may occur.
4-pole switching devices must be used if two TN-S subsystems are connected to each other. In TN-S systems, only one earthing bridge may be active (see Fig. 5/9 for coupling distributed feed-ins and in case of separate distributions). Therefore, it is not permitted that two earthing bridges be interconnected via two conductors.
Fig. 1/12: Multiple feed-in for low-voltage main distribution with central earthing point (CEP)
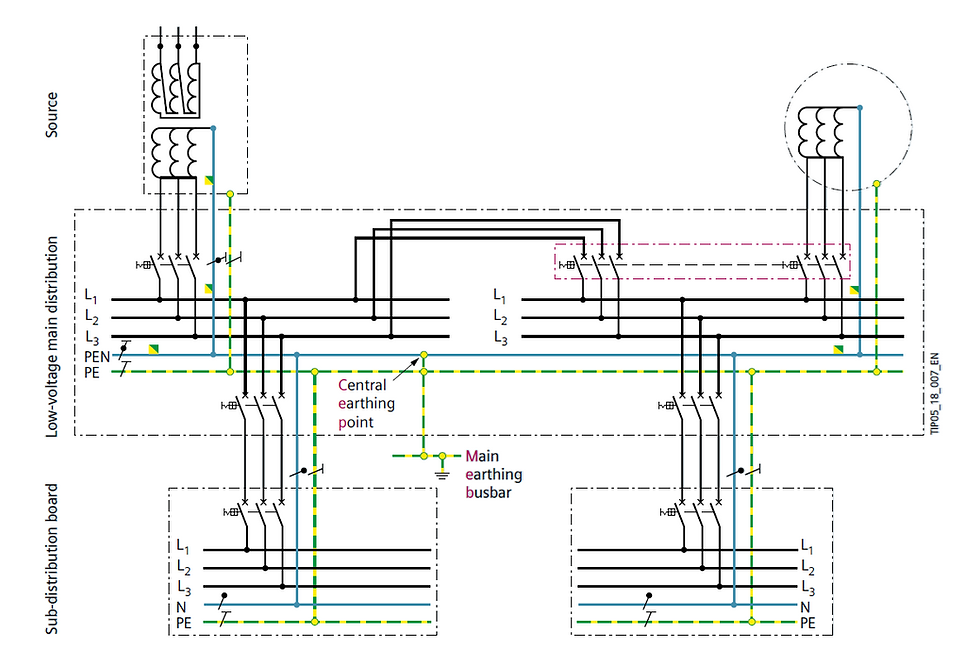
Today, networks with TT systems are only used in rural supply areas and in few countries. In this context, the stipulated independence of the earthing systems must be observed. In accordance with IEC 60364‑5‑54 (VDE 0100‑540), a minimum clearance ≥ 15 m is required.
Networks with an IT system are preferably used for rooms with medical applications in accordance with IEC 60364‑7‑710 (VDE 0100‑710) in hospitals and in production, where no supply interruption is to take place upon the first fault, for example in the cable and optical waveguide production. The TT system as well as the IT system require the use of residual current-operated protective devices (RCDs) – previously named FI (fault current interrupters) – for almost all circuits.
Tab. 1/13 provides a first approach for evaluating the different network systems depending on design and planning parameters. For project-related assessment, individual features can be selected and weighted.
Tab. 1/13: Exemplary quality rating dependent on the network system according to its type of connection to earth

Document: | Technical Principles - Planning of Electric Power Distribution by Siemens |
Format: | |
Size: | 13.24 MB |
Pages: | 374 |
Download: |
Comments